Revolutionizing plastic waste management through biological upcycling
Innovative research transforms plastic waste into valuable chemicals, paving the way for a circular economy and sustainable space travel
La Jolla, California—January 31, 2025—It is commonly understood that plastic waste is a tremendous problem. But how big of a problem? According to the U.N. Environment Programme, the world currently produces around 400 million metric tons of plastic waste per year—a number that is so far beyond human scale it is difficult to grasp. In terms of weight, yearly plastic waste is equivalent to about 73 million elephants or 40,000 Eiffel Towers.
A significant portion of this waste is mismanaged, meaning it is not recycled, it is incinerated, or it is stored in landfills. Estimates suggest that one to two million metric tons of plastic enter the oceans annually, some of which ends up in our food supply.
JCVI Professor Tae Seok Moon, Ph.D. has been long focused on addressing this problem, which he sees as an opportunity. Dr. Moon remarked, “Plastic waste is full of captured potential. We simply need to develop the tools to unlock it. By combining organisms that have the basic machinery needed to break down plastic coupled with advances in biological engineering, we can convert waste into useful products at scale.”
The circular economy
Broadly, Dr. Moon’s work is part of building a “circular economy” around plastic. The circular economy is a model of resource production and consumption that aims to eliminate waste and pollution by designing, reusing, and regenerating materials and products for as long as possible. This approach contrasts with the traditional linear economy, which follows a "take, make, and dispose" model.
In a recent paper that appeared in the journal Trends in Biotechnology, titled “Producing multiple chemicals through biological upcycling of waste poly(ethylene terephthalate),” Dr. Moon’s lab lays out a framework for bringing plastic waste into the circular economy.
Specifically, the paper addresses a type of plastic called polyethylene terephthalate (PET), commonly used in packaging, such as beverage bottles and food containers. PET is one of the most produced plastics, accounting for about 10% of total plastic production worldwide.
The paper details the development of the bacterium Rhodococcus jostii PET (RPET) as a microbial chassis for biological upcycling of post-consumer PET waste. The study introduces new genetic tools for RPET for genome editing.
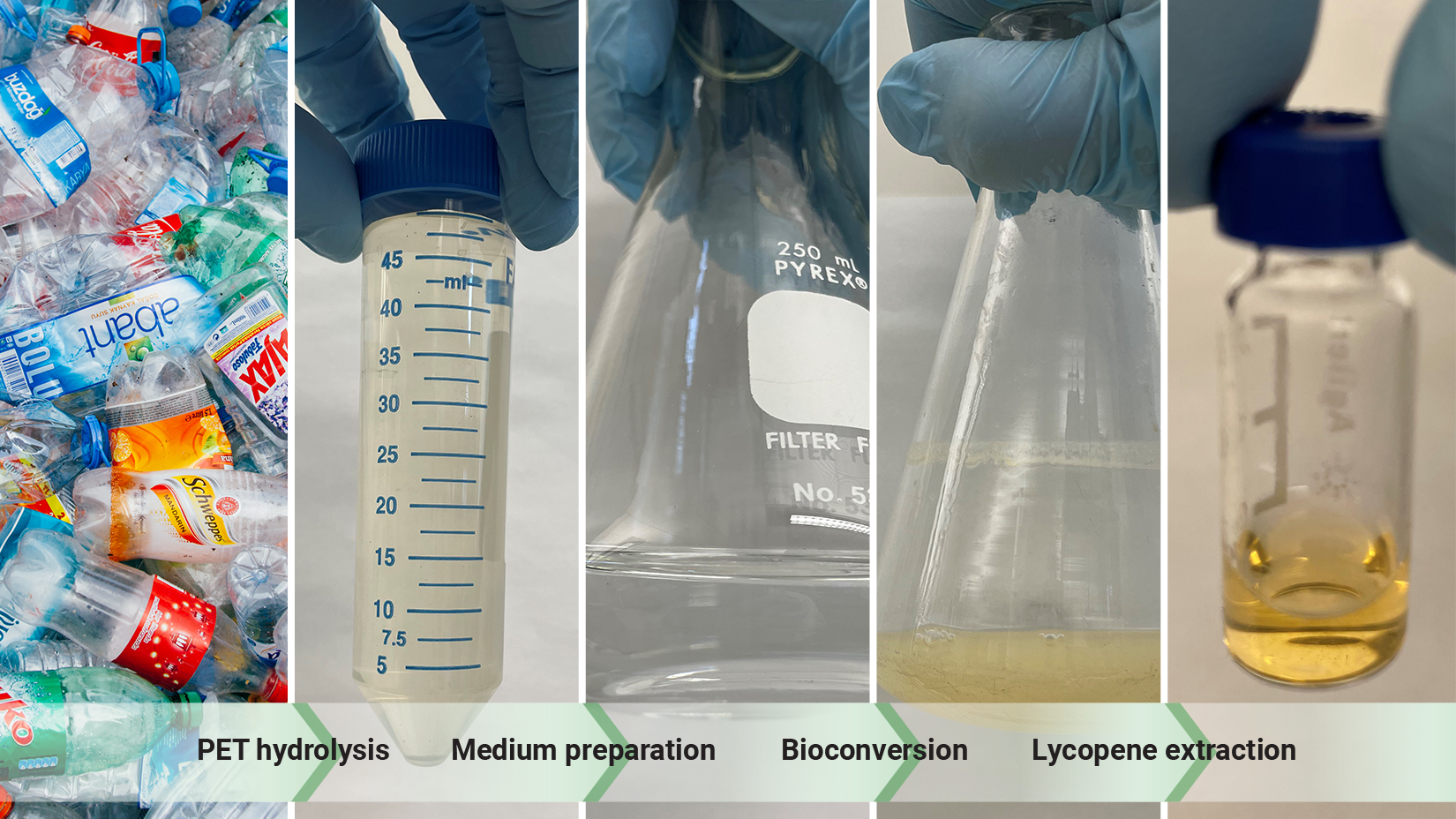
Using these tools, the researchers successfully engineered RPET to produce multiple valuable chemicals, including lycopene, lipids, and succinate, from PET waste, achieving high yields in fermentation processes.
The chemicals produced are of significant commercial value. Lycopene is what gives tomatoes their distinctive red color and is a powerful antioxidant. It is also used by the cosmetics industry as a pigment. Lipids can be used as a feed additive for animals, and in cosmetics, biolubricants, and biofuels. Succinate is used in the production of biodegradable polymers, solvents, and as a precursor for various industrial chemicals.
Adapting to space
While the challenges of waste management and inefficiencies in resource management are huge on Earth, they are acute in the context of human space travel. In space, resources are extremely limited and transferring additional resources to support space missions may be economically prohibitive or simply not possible.
Becoming extremely efficient with waste management may be the only way to overcome some of the logistical challenges of long-duration space travel. Dr. Moon’s lab has outlined bringing concepts developed on Earth to space travel and off-Earth destinations, like the Moon and Mars. Details from this work are found in “Developing an alternative medium for in-space biomanufacturing,” in the journal Nature Communications.
This paper introduces and validates a new biomanufacturing process called alternative feedstock-driven in-situ biomanufacturing (AF-ISM) that aims to reduce reliance on Earth-based resupply for long-duration space missions by utilizing readily available resources and waste streams to produce essential consumables.
As with the previous study, the workhorse is the bacterium RPET, which is ideal as it is self-sustaining, can self-replicate, has low energy demands, and is extremely adaptive. Similar to the previous study, Dr. Moon’s team focused on PET as its plastic feedstock. Additionally, they introduced two additional components to the feedstock: human waste and regolith. Regolith is a layer of loose, heterogeneous material covering solid rock, including dust and broken rocks, found on Earth, the Moon, Mars, asteroids, and other terrestrial bodies.
On human space flights, human waste is typically stored and disposed of on Earth. Using this waste solves several problems, including the challenge of waste storage and makes available energy and rich raw materials in a constrained environment. Using the AF-ISM process in simulated microgravity conditions, PET, human waste, and regolith were converted into a similar set of chemicals as the previous study in nearly the same amounts. PET serves as the carbon source, the human waste as the source of nutrients, and the regolith as the source of minerals for RPET to work its magic.
The road ahead
Both studies demonstrate promising scalability and economic feasibility for upcycling waste materials into valuable chemicals. The use of RPET in both studies shows potential for large-scale implementation. The processes have been validated in laboratory environments and are ready for further scaling up.
Techno-economic analysis reveals significant cost reductions and economic viability, especially for in-space biomanufacturing using alternative feedstocks like Martian and Lunar regolith, postconsumer PET, and fecal waste. The co-production of multiple chemicals enhances the economic competitiveness of these processes.
Addressing remaining challenges, such as optimizing feedstock formulations and resolving genetic modification issues, will be crucial for successful commercialization. Dr. Moon’s team is already working on these next steps through the newly formed Center for Innovative Recycling and Circular Economy (CIRCLE), one of six new NSF Global Centers focused on advancing bioeconomy research to solve global challenges.
Funding
Funding for these studies was provided by the U.S. Department of Energy (DE-SC0022003 and DE-SC0018324) and the Defense Advanced Research Projects Agency (HR001122S0010).
About J. Craig Venter Institute
The J. Craig Venter Institute (JCVI) is a not-for-profit research institute in Rockville, Maryland and La Jolla, California dedicated to the advancement of the science of genomics; the understanding of its implications for society; and communication of those results to the scientific community, the public, and policymakers. Founded by J. Craig Venter, Ph.D., JCVI is home to approximately 120 scientists and staff with expertise in human and evolutionary biology, genetics, bioinformatics/informatics, information technology, high-throughput DNA sequencing, genomic and environmental policy research, and public education in science and science policy. JCVI is a 501(c)(3) organization. For additional information, please visit www.jcvi.org.
Media Contact
Matthew LaPointe, mlapointe@jcvi.org, 301-795-7918